The Physics of Ocean Mapping: Picking the Right Tool for the Right Job
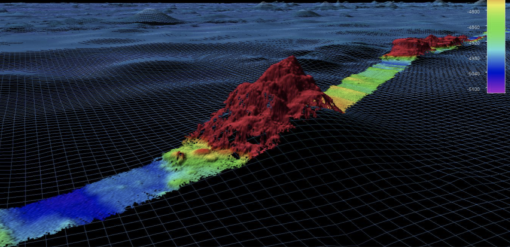
This blog was written by Science Communication Fellow Brandon Rodriguez.
Many people know the E/V Nautilus for the captivating videos taken from the depths of the ocean floor via remotely operated vehicles (ROVs) aboard. However, where we deploy those vehicles and the science we hope to conduct isn’t possible without an understanding of what lies below the waves.
Constructing maps of the ocean floor gives scientists insight as to what geography and geology are present in the ocean depths, guiding research to unseen ocean ridges, seamounts, and trenches. Yet our oceans remain largely unmapped, and the process of pulling back the curtain is a slow one. Our planet’s surface is over 70% water, representing nearly 225 million square kilometers (140 million square miles) of ocean floor. In order to better prioritize where to map and, in turn, where to dive next, we rely on complementary exploration techniques, each of which utilizes its own twist on the physics of waves.
Starting From the Sky
To begin, while true that we have very limited knowledge of the ocean floor, we actually have an incredibly complete picture of the ocean surface thanks to laser altimetry. Earth-orbiting satellites or aircraft with precisely known altitudes send laser pulses down to the ocean surface and measure the time it takes for that signal to reflect back to the instrument. Because light has a known and constant speed, we’re able to determine the ocean’s height to within just a few centimeters. Organizations like NASA and NOAA use altimetry to get complete maps of the ocean surface height every few days. With enough measurements, variability like water heights and tides can be averaged out.
While this doesn’t necessarily aid us in seeing what’s below the surface, the accuracy of the measurements allows us to observe local gravitational effects from large rock structures below. That’s because large structures like seamounts have greater mass than their surroundings and thus higher gravity, causing water to be more attracted and actually bulge above the average surface, with changes in heights on the order of tens of centimeters. If that height persists each time the altimeter passes, we know it’s not from local weather but from large gravity-inducing geography underneath.
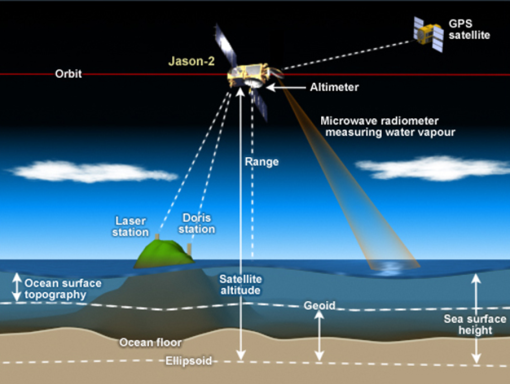
Using Light For a Deeper Understanding
While altimetry provides scientists with an idea as to where underwater features may exist, the resolution leaves much to be desired. The size of any hidden seamount needs to be enormous to induce a gravitational impact, and this doesn’t tell us anything about shape or complexity.
Scientists may turn to a second, but very similar, mapping technique called LIDAR, or light detection and ranging. Here, using a green light laser (normally with a wavelength of λ=532 nm), we can peer at best a hundred meters (over 300 feet) below the surface of the ocean. The green light is refracted as it passes through the water, diminishing the intensity of light that reaches the ocean floor and returns to the detector above. While the resolution is spectacular in the shallows, researchers are greatly limited by the depth that can be explored. As a result, LIDAR makes an excellent tool for shallow water and coastal mapping, but has very limited usage as we explore further from land.
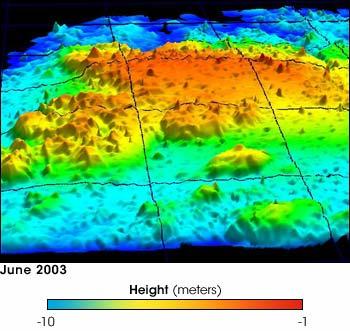
Obtaining a Clearer Picture
For any great depth of ocean, there is still no better tool than sonar. Sonar stands for SOund Navigation And Ranging, and as the name suggests, it measures the wave properties of sound rather than light. Light waves are transverse and travel best through the empty void of space where even air is missing, but sound waves are longitudinal and operate most effectively in denser water.
Because sound waves require movement of particles to propagate, we are at an advantage when mapping deeper water. Scientists and engineers carefully calibrate for factors within the water that affect the speed of sound as it travels to and from the ocean floor, such as temperature and salinity. Much like when using light, making these corrections give us a highly accurate measurement of the time needed for a signal to be sent to the surface below and then reflected back to the detector, providing incredibly high-resolution maps of the seafloor features.
Using multibeam sonar instead of a single pulse allows for scanning large swaths simultaneously, covering several kilometers in a single pass. Nautilus’s sonar is set up to map the seafloor at depths between 100 and 7,000 meters (300 to 23,000 feet) while cruising at ship speeds up to 12 knots (14 mph). In fact, engineers can even glean some information about the geology on the seafloor from the intensity, also known as backscatter, of the sound returned to the detector, as different surfaces tend to reflect or absorb sound in predictable ways; sound may get muffled when it hits a soft sandy floor versus that of a dense volcanic basalt.
In the end, using acoustic techniques like sonar provides the clearest picture in the most challenging of environments. While sonar requires the most time-intensive approach — namely a ship sailing overhead — it demonstrates how collaborative technologies contribute to ocean mapping. Each capable of boasting several pros and cons, but all relying on similar wave physics to guide ocean exploration.
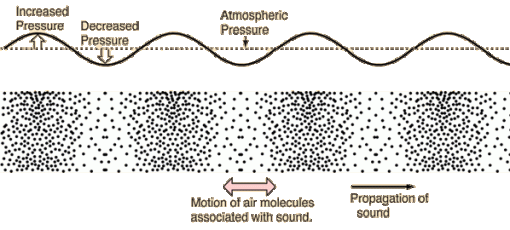
About the Author
Brandon Rodriguez has worked at JPL since 2016 in the K-12 education department, developing lessons for students and leading professional development training for educators. Before JPL, he originally was a research scientist, working at the Dow Chemical Company and in their alternative feedstocks platform. Brandon has taught high school and college chemistry and physics, as well as graduate-level science pedagogy and equity courses.
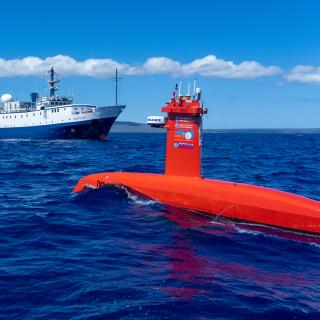
Luʻuaeaahikiikawawāapalaoa: Dual-Technology Seafloor Mapping
This expedition focuses on high-resolution mapping areas of Papahānaumokuākea Marine National Monument (PMNM) currently lacking data using ship-based mapping surveys in deep waters as well as deploying the uncrewed surface vessel DriX for nearshore mapping.